Rare diseases are classified according to their prevalence within a population. In the United States, the Rare Diseases Act of 2002 specifies that a rare disease impacts fewer than 200,000 individuals. Collectively, there are over 7,000 rare diseases affecting more than 30 million people. A significant number of these conditions are life-threatening, and the majority lack available treatments.1, 2 In contrast, the European Commission defines rare diseases as chronic or life-threatening conditions that impact fewer than 1 in 2,000 individuals, equivalent to approximately 50 per 100,000 people. An estimated 27 - 36 million people in the EU live with a rare disease, with around 6,000 to 8,000 distinct rare diseases identified.3
Epidemiology of Rare Diseases
The epidemiology of rare diseases reveals significant insights into their prevalence and impact:3-5:
- Approximately 80% of rare diseases have a genetic origin.
- About 70% of these diseases manifest during childhood, highlighting the importance of early diagnosis and intervention.
- Globally, rare diseases collectively affect an estimated 3.5% to 5.9% of the population, corresponding to approximately 263 to 446 million people worldwide.
- In Europe, between 6,000 and 8,000 distinct rare diseases affect millions, despite each condition being individually rare.
Most-Frequently Diagnosed Rare Diseases
While many rare diseases exist, some are more frequently diagnosed than others.
- Cystic Fibrosis: A hereditary condition that impacts the lungs and digestive system, most often found in people of European ancestry.
- Duchenne Muscular Dystrophy: A progressive and severe form of muscular dystrophy that predominantly affects males.
- Hemophilia: A genetic condition characterized by improper blood clotting, resulting in excessive bleeding.
- Gaucher Disease: A genetic disorder caused by an enzyme deficiency, leading to the accumulation of fatty substances in organs and tissues.
- Fabry Disease: A condition caused by a deficiency in an enzyme that leads to a buildup of fatty substances in various body tissues.
Cell and Gene Therapies for Treating Rare Diseases
Cell and gene therapies hold significant promise for treating rare diseases by targeting a disease’s genetic roots. With ongoing research and clinical trials, these therapies could transform the treatment landscape for many patients suffering from conditions previously deemed untreatable.Gene Therapy Approaches:
Gene therapy is a medical approach that treats or prevents diseases by introducing, modifying, or removing genetic material within a patient’s cells. These are particularly effective for rare diseases because nearly 80% are monogenic, meaning they are caused by mutations in a single gene. Gene therapy can provide long-term benefits or even a potential cure with a single treatment, contrasting sharply with traditional therapies that often require ongoing administration:
Methods of Gene Delivery:
In Vivo: Delivery of therapeutic genes directly into the patient’s body using vectors (e.g., viruses).
Ex Vivo: This method involves modifying cells outside the body and then reintroducing them into the patient. It is frequently employed in the treatment of hematological disorders. Two examples of gene delivery treatments are:
- Luxturna: Treats biallelic RPE65 mutation-associated retinal dystrophy.
- Zolgensma: Approved for spinal muscular atrophy, demonstrating significant efficacy in restoring motor function.
Cell Therapy Approaches:
Various diseases can be successfully treated by using living cells. This technique is called cell therapy and can include:
- Stem Cell Therapy: Utilizes stem cells to regenerate damaged tissues or correct genetic defects. For instance, doctors can now generate induced pluripotent stem cells (iPSCs) from a patient’s somatic cells, which are corrected for mutations and then differentiated into specific cell types for transplantation.
- CAR T-cell Therapy: A type of immunotherapy that modifies T-cells to better target and destroy cancer cells. This therapy has shown promise in treating rare cancers.
Gene and cell therapies are advancing continuously, with ongoing research focused on enhancing their effectiveness and making them more accessible. Despite challenges related to their complexity and high development costs, steady progress is being achieved.
The Role of Real-World Data in Rare Diseases
Real-world data (RWD) is pivotal in overcoming barriers that limit access to cell and gene therapies for rare diseases. It enables more effective development and implementation of these innovative treatments by providing comprehensive insights into disease characteristics, supporting health technology assessment (HTA) processes, identifying specific patient populations, informing clinical trial designs, and enhancing post-marketing surveillance. As regulatory bodies increasingly recognize the value of RWD, its role in facilitating access to life-saving therapies is likely to grow even further.
Understanding Disease Progression and Patient Needs:
Real-world data helps characterize the natural history of rare diseases, informing developers about disease progression, symptom severity, and patient demographics. This understanding is vital for designing effective therapies and clinical trials.6 For example, in developing therapies for spinal muscular atrophy (SMA), RWD has been instrumental in identifying the most affected populations and understanding the disease's impact on quality of life. This information guided the approval process for Zolgensma, which targets the underlying genetic cause of SMA.
Supporting Health Technology Assessments:
Real-world data provides evidence that can support HTA decisions, which are critical for determining coverage and reimbursement for new therapies. Given the challenges of traditional trial data in rare diseases, RWD offers a complementary perspective.7 For example, the approval of Cerliponase alfa for Batten disease was based on a single-arm trial compared to historical controls derived from natural history registries. This approach allowed regulators to assess treatment effectiveness in a real-world context, facilitating earlier access to the therapy.
Identifying Patient Populations:
Real-world data can help identify specific patient populations that may benefit most from certain therapies, optimizing treatment strategies and resource allocation.8 For example, in gene therapy trials for sickle cell disease, RWD highlighted variations in treatment response among different ethnic groups. This knowledge has influenced the design of more inclusive clinical trials and targeted therapies, such as Exa-Cel, which recently received marketing authorization.
Informing Clinical Trial Design:
Integrating RWD into clinical trial design can enhance efficiency by identifying appropriate endpoints, patient selection criteria, and potential confounding factors. Modeling approaches that incorporate RWD have been applied in trials for gene therapies targeting hemoglobinopathies. These models help predict responses to treatment and optimize trial designs, ultimately accelerating the development process.9
Enhancing Post-Marketing Surveillance:
Once therapies are approved, RWD is essential for ongoing long-term safety and efficacy monitoring. This data can inform regulatory decisions and support continued access to treatments. RWD collected from patients has provided insights into real-world outcomes, helping to refine treatment protocols and identify any emerging safety concerns, as in several cases of gene therapies like Luxturna for inherited retinal dystrophies.10
Using Registries to Generate Evidence on Rare Diseases:
Registries are databases that provide insights into the epidemiology of rare or ultra-rare conditions. They can support orphan drug applications and aid in characterizing clinical phenotypes and genotypes.11 Registries serve as a valuable source of RWD for addressing particular challenges in rare disease research. Their primary advantage lies in their higher likelihood of accurately capturing diagnoses compared to other RWD sources like electronic medical records or administrative databases.
Registries can be categorized into three main types: patient registries, disease registries, and product registries. Patient registries are designed to recruit individuals with rare diseases and may encompass multiple rare conditions within a single registry. Disease registries, a subset of patient registries, concentrate on specific diseases. Product registries, on the other hand, are centered around particular medications. These registries can be used for research mandated by regulatory authorities and are usually established by pharmaceutical companies to monitor the usage of specific drugs or created for purposes of public health surveillance.
Barriers and opportunities for access to gene therapies:
Patient access to these innovative therapies is not straightforward. In October 2022, Pfizer and the Alliance for Regenerative Medicine convened a roundtable at the World Health Summit in Berlin, Germany, with representatives across stakeholder groups, including academia, civil society, industry, patient communities, healthcare professionals (HCPs), and governments, to discuss these topics. A summary of barriers and opportunities to accessing gene therapies was identified at the roundtable and is presented in the table below:12,13
Barriers to Gene Therapies |
Ways to Address Barriers |
Lack of infrastructure for diagnosis and determining patient eligibility |
|
Lack of infrastructure for gene therapy delivery |
|
Obstacles to the appropriate value assessment of gene therapies and the adoption of innovative financing models
|
|
Case Study
Axtria collaborates closely with pharmaceutical companies and key opinion leaders to create tailored, innovative, clinically validated, and scientifically rigorous solutions that tackle complex challenges. The RWE/HEOR/ES team at Axtria leverages its deep expertise in rare diseases alongside advanced AI/ML technologies to deliver impactful outcomes. Axtria’s latest blog, Modeling the "Difficult to Model" Disease: Considerations for Evaluating the Cost-Effectiveness in Rare Diseases, highlights innovative approaches addressing challenges in cost-effectiveness modeling for rare diseases. In a recent project, Axtria utilized lab data linked to claims to identify and assess clinical and economic outcomes among patients with a rare disease that has not been assigned a diagnosis code.
Situation: Congenital thrombotic thrombocytopenic purpura (cTTP) is a rare condition categorized under thrombotic microangiopathy (TMA). It results from a significant lack of the von Willebrand factor cleaving enzyme, a disintegrin and metalloprotease with thrombospondin type 1 repeats, member 13 (ADAMTS13), with a likely diagnosis made when ADAMTS13 activity drops below 10%. However, there is limited and inconsistent evidence linking ADAMTS13 activity to clinical outcomes, such as relapse rates and mortality in cTTP patients. Additionally, identifying cTTP patients was challenging due to the lack of a diagnosis code in the ICD-10, a well-known system for cataloging health conditions. We used laboratory results for ADAMTS13 activity, antibody testing, and medical claims data to classify patients and assess their clinical profiles, trajectories, healthcare resource utilization, and symptom burden.
Axtria conducted a study to quantify the disease burden of cTTP and examine how the ADAMTS13 enzyme affects clinical outcomes. In anticipation of a new gene editing therapy designed to enhance ADAMTS13, the client wanted to explore the correlation between ADAMTS13 activity levels and patient outcomes, in addition to healthcare resource utilization.
Approach: The study consisted of two stages. First, we collaborated with key opinion leaders to conduct a feasibility assessment for identifying patient cohorts, including likely cTTP, likely immune-mediated thrombotic thrombocytopenic purpura ( iTTP), and other TMAs, based on A13 activity levels, antibody tests, treatment trajectories, and other relevant stratifications with linked claims and labs data.
We employed descriptive statistics to report the number of patients with lab results, testing frequency, and longitudinal changes in ADAMTS13 levels. Endpoints were also captured in counts and percentages, stratified by varying activity levels. The second stage focused on statistical analysis for the association between ADAMTS13 activity levels and clinical symptoms or outcomes, encompassing demographic and clinical characteristics of cTTP patients, associated symptoms and healthcare resource utilization (HCRU). Correlation analysis and Bayesian network analysis helped us understand associations, while multivariable regression modeling quantified the relationship between ADAMTS13 activity as the main predictor and clinical outcomes.
Results: The proportion of likely diagnoses, sex distribution, and average ADAMTS13 activity levels were consistent with expectations based on clinical literature. The mean ADAMTS13 activity for the likely cTTP cohort was approximately 15.3%, the likely iTTP cohort had a mean of 34.9%, and the other TMA cohort averaged 48.9%. Additionally, we observed that all-cause HCRU and bleeding symptoms were more frequent among patients with lower average ADAMTS13 activity (≤ 30%). Furthermore, all-cause overall HCRU was notably higher in patients who exhibited greater variability (SD >10) in their ADAMTS13 activity levels over time.
Key takeaways:
- Compared to genetic testing, our novel study of rare disease patient identification using linked claims and lab data was cost-efficient for confirming cTTP diagnoses.
- Evidence generation supported the client value message that a lower ADAMTS13 activity level is associated with higher HCRU.
Conclusion
The absence of robust data on the impact of rare diseases, their progression, patient characteristics, and treatment outcomes, coupled with stringent policies, has considerably delayed the approval and reimbursement of new drugs targeting these conditions. As more cell and gene therapies gain regulatory approval globally, it is essential to prioritize timely access to these innovative treatments for individuals with rare genetic disorders.
Because of its ability to identify patient populations, support adaptive trial designs, and enhance regulatory approval processes, RWD holds the potential to be pivotal in accelerating access to rare disease cell and gene therapies. It enables post-market surveillance and demonstrates long-term treatment benefits. Registries serve as a valuable source of RWD for addressing particular challenges in rare disease research. Their primary advantage lies in their higher likelihood of accurately capturing diagnoses compared to other RWD sources like electronic medical records or administrative databases. Registries are becoming increasingly significant in regulatory contexts.
It is essential to consider the actions health systems must take to ensure access. Adopting a patient-centered approach is crucial, as engaging patients in the development process is key to designing therapies that effectively address their needs. Strong collaboration among all stakeholders, healthcare professionals, patient advocacy groups, policymakers, biopharmaceutical companies, and payers is essential.
Appendix: List of U.S. FDA-Approved Cell and Gene Therapies
As of December 2024, 43 cell and gene therapy products have been approved by the U.S. FDA.14 Links to each product are included below:
|
Product and Trade Name |
Manufacturer |
1 |
Celgene Corporation, a Bristol-Myers Squibb Company |
|
2 |
Ferring Pharmaceuticals A/S |
|
3 |
SSM Cardinal Glennon Children's Medical Center |
|
4 |
Iovance Biotherapeutics, Inc. |
|
5 |
Autolus Limited |
|
6 |
Pfizer, Inc. |
|
7 |
Juno Therapeutics, Inc., a Bristol-Myers Squibb Company |
|
8 |
Janssen Biotech, Inc. |
|
9 |
Vertex Pharmaceuticals Incorporated |
|
10 |
Cleveland Cord Blood Center |
|
11 |
Duke University School of Medicine |
|
12 |
Sarapeta Therapeutics, Inc. |
|
13 |
GINTUIT (Allogeneic Cultured Keratinocytes and Fibroblasts in Bovine Collagen) |
Organogenesis Incorporated |
14 |
New York Blood Center |
|
15 |
CSL Behring LLC |
|
16 |
Clinimmune Labs, University of Colorado Cord Blood Bank |
|
17 |
MD Anderson Cord Blood Bank |
|
18 |
LifeSouth Community Blood Centers, Inc. |
|
19 |
Bloodworks |
|
20 |
BioVex, Inc., a subsidiary of Amgen Inc. |
|
21 |
PTC Therapeutics |
|
22 |
Novartis Pharmaceuticals Corporation |
|
23 |
CellTrans Inc. |
|
24 |
Fibrocell Technologies |
|
25 |
Orchard Therapeutics (Europe) Limited |
|
26 |
Spark Therapeutics, Inc. |
|
27 |
bluebird bio, Inc. |
|
28 |
MACI (Autologous Cultured Chondrocytes on a Porcine Collagen Membrane) |
Vericel Corp. |
29 |
Gamida Cell Ltd. |
|
30 |
Dendreon Corp. |
|
31 |
StemCyte, Inc. |
|
32 |
Enzyvant Therapeutics GmbH |
|
33 |
BioMarin Pharmaceutical Inc |
|
34 |
Mesoblast, Inc. |
|
35 |
Humacyte Global, Inc. |
|
36 |
bluebird bio, Inc. |
|
37 |
STRATAGRAFT (allogeneic cultured keratinocytes and dermal fibroblasts in murine collagen-dsat) |
Stratatech Corporation |
38 |
Kite Pharma, Inc. |
|
39 |
Adaptimmune LLC |
|
40 |
Krystal Biotech, Inc. |
|
41 |
Kite Pharma, Incorporated |
|
42 |
bluebird bio, Inc. |
|
43 |
Novartis Gene Therapies, Inc. |
References
- Rare diseases at FDA. United States Food and Drug Administration. Accessed January 14, 2025. https://www.fda.gov/patients/rare-diseases-fda
- Medical products for rare diseases and conditions. United States Food and Drug Administration. Accessed February 26, 2024. https://www.fda.gov/industry/medical-products-rare-diseases-and-conditions
- Rare diseases at the European Commission. Accessed January 14, 2025. Rare diseases - European Commission
- Chung CCY; Hong Kong Genome Project; Chu ATW, Chung BHY. Rare disease emerging as a global public health priority. Front Public Health. 2022 Oct 18;10:1028545. doi: 10.3389/fpubh.2022.1028545. PMID: 36339196; PMCID: PMC9632971.
- Nguengang Wakap, S., Lambert, D.M., Olry, A. et al.Estimating cumulative point prevalence of rare diseases: analysis of the Orphanet database. Eur J Hum Genet 28, 165–173 (2020). https://doi.org/10.1038/s41431-019-0508-0
- Liu J, Barrett JS, Leonardi ET, Lee L, Roychoudhury S, Chen Y, Trifillis P. Natural History and Real-World Data in Rare Diseases: Applications, Limitations, and Future Perspectives. J Clin Pharmacol. 2022 Dec;62 Suppl 2(Suppl 2):S38-S55. doi: 10.1002/jcph.2134. PMID: 36461748; PMCID: PMC10107901.
- Dayer VW, Drummond MF, Dabbous O, Toumi M, Neumann P, Tunis S, Teich N, Saleh S, Persson U, von der Schulenburg JG, Malone DC, Salimullah T, Sullivan SD. Real-world evidence for coverage determination of treatments for rare diseases. Orphanet J Rare Dis. 2024 Feb 7;19(1):47. doi: 10.1186/s13023-024-03041-z. PMID: 38326894; PMCID: PMC10848432.
- Benjamin A. Derman, Andrew J. Belli, Minoo Battiwalla, Mehdi Hamadani, Ankit Kansagra, Hillard M. Lazarus, Ching-Kun Wang, Reality check: Real-world evidence to support therapeutic development in hematologic malignancies, Blood Reviews, Volume 53, 2022, 100913, ISSN 0268-960X, https://doi.org/10.1016/j.blre.2021.100913.
- Thomas A. Fox, Claire Booth; Improving access to gene therapy for rare diseases. Dis Model Mech1 June 2024; 17 (6): dmm050623. doi: https://doi.org/10.1242/dmm.050623
- Fischer MD, Simonelli F, Sahni J, Holz FG, Maier R, Fasser C, Suhner A, Stiehl DP, Chen B, Audo I, Leroy BP; PERCEIVE Study Group. Real-World Safety and Effectiveness of Voretigene Neparvovec: Results up to 2 Years from the Prospective, Registry-Based PERCEIVE Study. Biomolecules. 2024 Jan 17;14(1):122. doi: 10.3390/biom14010122. PMID: 38254722; PMCID: PMC10813228.
- Pisa, F., Arias, A., Bratton, E., Salas, M., & Sultana, J. (2023). Real world data for rare diseases research: The beginner’s guide to registries. Expert Opinion on Orphan Drugs, 11(1), 9–15. https://doi.org/10.1080/21678707.2023.2241347.
- Bhatia S, Le Cam Y, Carrion J, Diamond L, Fennessy P, Gassman S, Gutzwiller F, Kagan S, Pankevich D, Young Maloney J, Mahadev N, Schulz M, Wong-Rieger D, Morgese P. Strengthening health systems for access to gene therapy in rare genetic disorders. Mol Ther Methods Clin Dev. 2024 Mar 13;32(2):101220. doi: 10.1016/j.omtm.2024.101220. PMID: 38516694; PMCID: PMC10951444.
- A Framework to Strengthen Health Systems for Gene Therapy for Rare Genetic Diseases. Accessed January 14, 2025. A-Framework-To-Strengthen-Health-Systems-for-Gene-Therapy_5.23.23.pdf
- S. FDA Approved Cellular and Gene Therapy Products. Accessed January 14, 2025. Approved Cellular and Gene Therapy Products | FDA
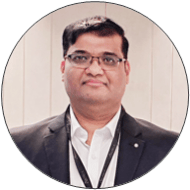